In the search for life in the Galaxy, an important question to ask is: Can we predict which parts of galaxies are more likely to have habitable planets? If we knew the answer, we could target our search at the right parts of the sky, and we could assess the odds for life appearing in galaxies throughout the cosmos.
In an attempt to answer this question, the concept of the Galactic Habitable Zone was established. This is built from considering two factors that affect habitable planets: the availability of raw materials for planet construction (what astronomers refer to as metallicity, or “everything that isn’t hydrogen and helium”), and the chances of being hit by high levels of damaging ultraviolet radiation.
The Earth’s atmosphere screens the worst of the Sun’s ultraviolet rays, but if we were swamped by radiation from say a nearby supernova, that radiation could deplete the ozone layer, and cause a mass extinction. Some astronomers and geologists think there is some evidence that Earth’s geological and biological history bears the scars of such supernovae, but this is far from clear.
These two factors have a common link: star formation. Most of the heavy elements which make up planets like Earth, and all the life-forms along for the ride, are forged in the cores of stars. The elements heavier than iron are born in supernovae and other massive explosions that occur at the end of a massive star’s life. So local star formation is a blessing and a curse for habitable planets: we need several generations of star birth and death to give us the ingredients for planets, but vigorous star formation results in supernovae which can be deadly if too close.
In the classic galactic habitable zone picture, this means that the interior of galaxies are ruled out because the local radiation is too hazardous, and the outer parts are too metal poor to form habitable planets. Somewhere in the middle, things are best, a Goldilocks zone that is reminiscent of the Goldilocks zone around stars (although they exist for quite different reasons). The Galactic Habitable Zone is therefore an annulus, a ring around the Galaxy’s centre, which the Sun happens to reside in.
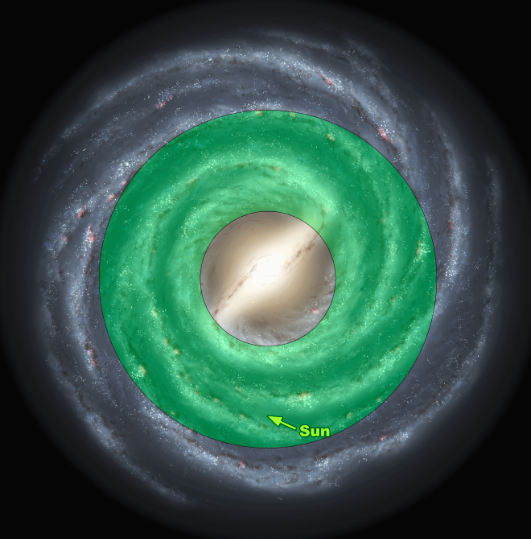
However, this classical picture assumes galaxies are nice geometric shapes. And they ain’t. They grow through collisions with other galaxies, and tear up satellite galaxies into streams of stars and gas before gobbling them up entirely.
So in our latest paper, we tried to look at how this messy picture of galaxy formation affects the galactic habitable zone. We took a cosmological simulation of the Local Group, our intergalactic neighbourhood which includes The Milky Way, Andromeda and Triangulum galaxies. These simulations model the dark matter distribution on large scales, and the gas on small scales, with some of the gas becoming dense enough to form stars, which can then enrich the surrounding gas with heavy elements when they die. We took the data from these simulations, and used standard galactic habitability criteria to measure the GHZ.
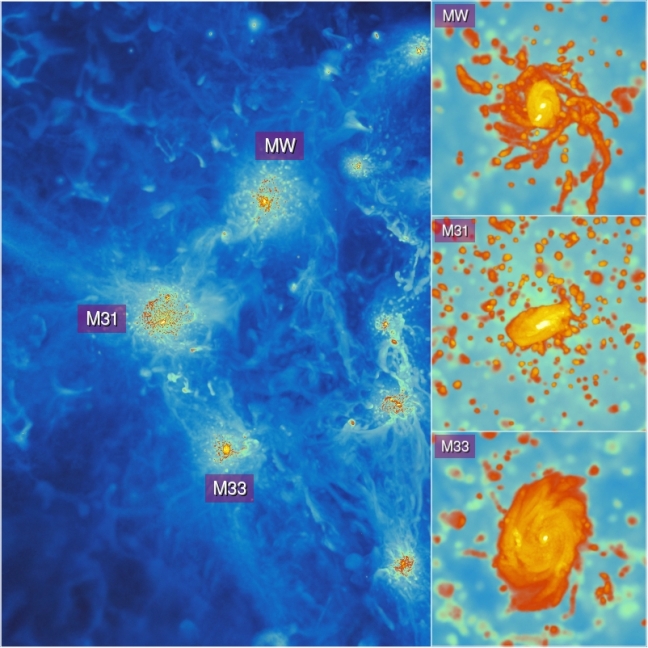
And it’s quite a mess! The GHZ isn’t nice and symmetric at all. Those big streams of stars and gas I mentioned? Quite suitable for habitable planets apparently. As are those satellite galaxies before they’re gobbled up. The best places to live in galaxies shifts quite a bit over time, as the whole edifice is assembled through all those collisions and mergers. Each galaxy has its own path to tread towards producing habitable planets.

In the animation above, it looks like the very centre is the most habitable, but really the centre has the highest concentration of stars. Each star has far less habitable planets, but this makes a lot of habitable planets if we cram enough stars together. The best spots are further away from the centre typically, but this changes quite a bit as the galaxy is built.
But this is only really the beginning. What we discovered in this work is how inadequate our understanding of the problem really is. We still have lots of questions about how supernovae can sterilise a planet, and we haven’t made a big enough census of exoplanets to really nail down the relationship between metallicity and producing habitable planets. There are other things that can make parts of the Galaxy uninhabitable, which we weren’t able to look at in this paper – if stars are too crowded together, they can knock habitable planets away from their host (which would make the galactic centre very bad); gamma ray bursts can explode much more powerfully than supernovae, with potentially more devastating results.
What we do know is that whatever the true Galactic Habitable Zone of the Milky Way is, it ain’t an annulus.